Boron Carbide (B4C)
Boron carbide is one of the hardest known materials being only second to diamond. Its composition ranges from B4.3C to B10.4C. Technical boron carbide usually has a high carbon content and is labelled B4C, for convenience. Due to its high hardness and Young’s modulus it is very resistant against abrasion and thus used in blast or spray nozzles and as an abrasive. These properties combined with its low density make B4C a superior material for light-weight ballistic armor [SCH15].
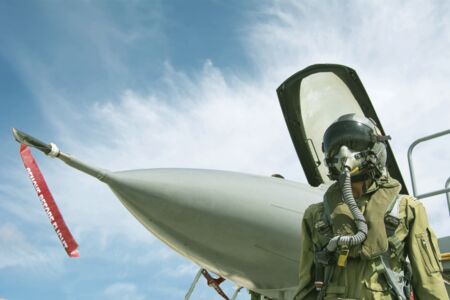
It is particularly noteworthy that the hardness is retained even at high temperatures, so that boron carbide above 1000 °C, the hardest known material is [Hül14]. It should be noted, however, that use above 1000 °C in air is not appropriate, as reactions at the surface result in continuous decomposition of the material [Li07]. As early as 500 to 600 °C, the boron carbide forms an oxide layer within a very short time, in which cracks subsequently form only by the cooling process [Li07] [Sal07]. In damp air, a hydrogen- and oxygen-containing surface layer is formed [Sal07]. Even in the absence of an oxidizing atmosphere, the material does not remain stable up to the melting point of 2600 °C, but exhibits decomposition reactions at the surface from 1800 °C [Jim98].
Boron carbide is used in particular in areas where high hardness with low component weight is required [Red13]. The density is only 2.5 g / cm3 [Red13]. Anti-shocking is therefore a typical application [Sal07] [Che03]. In addition, there is the application in the field of nuclear technology, as boron carbide is an excellent neutron absorber [Laz99]. The high hardness makes the material ideal for all applications where tribological stress prevails [Laz99]. Boron carbide is therefore used for linings of mortars and ball mills [Sal07]. In addition, boron carbide is used for boriding steels. The resulting surface-borne egg-boride compounds give the steel better resistance to friction and chemical stress [Sch15]. Despite the outstanding properties of the material in terms of hardness and wear resistance, some limitations must be considered. A major challenge is the handling of the brittle behavior of the material and the low fracture toughness. It is possible by a higher compression to 95% of the theoretical density [Sal07] to mitigate this behavior, however, the necessary hotpressing process is very expensive [Hal89]. Another possibility is infiltration with a metallic melt [Hal89] or the admixture of, for example, TiB2 [Hül14] or Al4C3 [Sch15].
The high chemical reactivity of boron carbide with metals is responsible for the fact that the use as a cutting material is very limited despite the high hardness [Sal07]. From a certain projectile speed, the application as ballistic protection is also no longer possible, since the resistance to shear stress drops drastically as of a certain shock load due to microscopic phenomena [Che03] [Red13].
A peculiarity of boron carbide can be found on the chemical or crystallographic level. Boron carbide is present in the rhombohedral crystal lattice and the atoms form strong covalent bonds with each other, which promotes high hardness [Red13]. The lattice has a very high solubility, so that the composition of the material can fluctuate between B4C and B10C without a new phase being formed [Bal07].
In the case of boron carbide, high importance is attached to the manufacturing process. Their outstanding properties are demonstrated by the boron carbide components when a very fine and homogeneous microstructure is set in the manufacturing process [Hül14]. Since the diffusion coefficients of carbon and boron in the boron carbide are relatively low, press-sintering or hot-isostatic pressing can be used [Hül14] [Bre03]. As a result, the compression is also driven by pressure in addition to the thermally activated diffusion [Hül14]. The sintering process takes place at about 2000 °C. under an inert gas atmosphere [Bre03]. Pressure-sintered boron carbide does not have satisfactory mechanical properties due to the resulting coarser structure and residual porosity [Sal07].
The synthesis of boron carbide follows the principle of the Acheson process, which is used for the production of silicon carbide. Electric energy is introduced in an electric arc furnace or a resistance-heated furnace in order to drain the strongly endothermic reaction. It must be reached about 2500 °C in order to achieve a satisfying yield of boron carbide. The starting materials are boron oxide and petroleum coke, which are converted into boron carbide and carbon monoxide [Sal07]. Finally, the recovered boron carbide is ground to powder.
If finer crystals in the range of 0.1 to 1.5 microns are required, an addition of magnesium, which is removed afterwards in the form of magnesium oxide, makes sense [Sch15]. For even finer crystals, fabrication methods such as CVD or plasma-assisted methods are feasible, but at higher cost [Sch15].
Application Examples
Milling Tools, Neutron Absorber Material, Ballistic, Technical Ceramic-Nozzles, Cutting Tools, Wear Protection, for Cold Isostatic Pressing, Green Machining, Parts with Complex Geometry